The
Mystery of Quantum Physics (Part 2): Spooky Action at a Distance
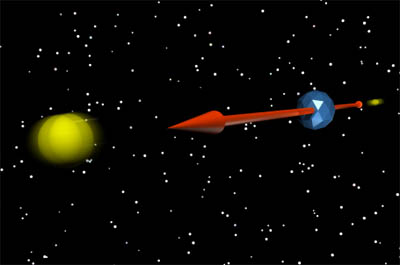
No
matter how far apart two entangled photons are - even light
years - they can affect each other instantly.
|
Last month we started our expedition into the
weird world of quantum physics. A place where things do not exist
unless you look at them, where cats can be both dead and alive
at the same time. This month we will focus on how some interpretations
of quantum physics suggest that everything in the universe is
instantly connected with everything else, no matter how distant
apart they are. (Note, it is strongly recommended that you read
part one before proceeding to part
two).
The year 1927 saw the start of a series of debates
between two of the most knowledgeable scientists in the world
at the time: Einstein, the author of the theory of general relativity
and Neils Bohr, one of the early researchers in quantum theory.
The first clash took place at Fifth Solvay International Conference
on Electrons and Photons held in Brussels, Belgium. The attendees
at this conference were few, but distinguished. Of the 29 scientists
there 17 had, or would in the future, win a Nobel Prize. Marie
Curie won two.
Einstein, despite being one of the founders of quantum
theory, was uncomfortable with it. One of Einstein's greatest
strengths as a scientist was his ability to conduct thought experiments
- experiments that might be impossible to do in real life, but
when done in the imagination yielded clues to the nature of physics.
(One of Einstein's most productive thought experiments was to
consider what the world would look like if he could ride his bike
at the speed of light). Attempting these kinds of thought experiments
to get at the true nature of quantum theory, however, was frustrating.
The results seemed nonsensical. Objects didn't appear to be there
unless you looked at them. Cats turned out to be both dead and
alive at the same time. If you knew where a particle (such as
an electron) was exactly, you couldn't tell anything about how
it was moving.
Bohr didn't have a problem with this, however. He
seemed unconcerned with the great conundrums that the theory raised,
only in what the results that the equations gave. As physicist
David Mermin once put it, Neil Bohr's attitude, as expressed in
his famous Copenhagen Interpretation of quantum physics, was just
'Shut up and calculate!'

The
attendees of the Fifth Solvay International Conference
on Electrons and Photons. Einstein is seated in the
first row towards the center.
|
A typical Einstein/Bohr encounter would start with
Einstein coming up with an example to show that quantum theory
was wrong or incomplete. Bohr would then spend the next evening
pondering the problem and reply the following day with something
to discredit Einstein's criticism. The debate came to a head in
1935 when Einstein, along with Boris Podolsky and Nathan Rosen,
published a paper that described what would become known as the
EPR paradox (for Einstein-Podolsky-Rosen paradox).
Spooky
Action at a Distance
Einstein's thought experiment in the paper consisted
of taking a particle (for our example let's use a pion) and letting
it decay into two photons (light particles) that race off in different
directions. Because they came from the same pion, the photons
are "entangled." This means that they share a common wave function.
The two photons would also have some complementary characteristics.
For example, their spin: The pion had no spin to begin with, so
if one photon is observed to have an up spin on its x-axis,
the other, to make things equal, must have an opposite down
spin on its x-axis.
According to quantum theory, however, the property
does not exist until it is measured. So when you measure the first
photon and find it has an up spin, that forces the other photon
to instantly assume a down spin even if the second photon might
be a light year away from the first. Einstein and his co-authors
argued that this didn't make sense. Either the photons had carried
the spin information with them when they first split up, or the
first photon, when it was measured, must have communicated its
spin to the second one instantly across an enormous difference
at faster than the speed of light. Einstein referred to this effect
as "spooky action at a distance."
Since information cannot travel faster than the
speed of light, this caused Einstein to argue that the photons
must have "hidden variables" that carried the spin information
since the pair of photons were created. Since quantum theory didn't
allow for these variables, the theory must be incomplete.
Bell
and his Theorem
Einstein's "spooky action at a distance" challenge
remained unresolved past both his and Bohr's deaths in 1955 and
1962 respectively. In 1964 an Irish physicist named John Bell
published a paper entitled On the Problem of Hidden Variables
in Quantum Mechanics. Bell initially supported Einstein and
thought that there must be hidden variables. In his paper he suggested
a test to see if hidden variables could account for what was being
seen.

Bohr
(left) and Einstein's (right) argument over
the ERP paradox wasn't settled until Bell came up with this
theorem and Clauer did the experiment showing that Bohr
was right.
|
Bell's test consisted of creating a pair of entangled
particles and sending them off to two people (traditionally known
as Alice and Bob) who then test the particles for a complementary
property like spin. The specifics are hard to understand, but
Bell was able to show that over a large number of trials, the
number of times that Alice and Bob's results agree should be different
if the properties were pre-existing than if they were being created
when the first one was measured as quantum theory says they are.
Bell thought that after he published his theorem (often referred
to as "Bell's Inequity" because of one of its predictions) it
would be many years before anybody could set a up test to actually
try it. Only a year later, however, an enterprising graduate student
at Columbia University, John Clauser, was able to run a crude
version of the experiment and showed that the photons obeyed what
was predicted by quantum physics, not what would be expected from
a hidden variables theory. Another scientist, Alan Aspect, later
duplicated Clauser's experiments with greater accuracy proving
that despite Einstein's qualms, there was indeed "spooky action
at a distance" in the quantum world.
Bell's work opened up what was largely thought to
be a philosophical issue to experimentation. His contribution
was so great that Henry Stapp of the Lawrence Berkeley National
Laboratory in California was later to call Bell's work on quantum
theory "the most profound discovery of science".
Bohm's
Interpretation
Despite his work proving quantum theory's accuracy,
Bell was not a fan of the standard Copenhagen Interpretation with
its dependency on observation to collapse the wave function and
make a particle (or for that matter a cat) real. Bell found an
interpretation created by physicist David Bohm taht made more
sense to him. To understand Bohm's interpretation, it is helpful
to go back to our example in part one of looking at the star Alnilam
in the constellation Orion. In our discussion of the Copenhagen
interpretation we saw that a photon, a particle of light, doesn't
really leave Alnilam. Instead, a wave of probability makes
the trip to our eyes. In Bohm's interpretation there is indeed
a real photon that leaves the star guided by a "quantum potential"
force that moves backwards in time like a beacon to bring the
particle to us. Everything in the universe is connected in Bohm's
interpretation. There is no need for a wave function to collapse
on observation as needed in the Copenhagen interpretation. However,
this interpretation isn't without its problems. While it is deterministic,
which means that with enough information you can absolutely predict
everything that will happen in the universe from the very beginning,
it requires information to travel backwards in time and instantly
across immense distances. For these reasons the Bohm interpretation
has not had a wide following among scientists.
The
"Many Worlds" Interpretation

In
the Many Worlds interpretation the universe would split
and Schrödinger's cat would be dead in one and alive in
the other. (Credit Dc987 and Wikipedia
Commons)
|
Perhaps the most noteworthy alternative to the standard
Copenhagen interpretation among physicists that study quantum
theory is entitled the "Many Worlds" interpretation. Such notable
scientists as Stephen Hawking and Richard Feynman have supported
the interpretation and it seems to be getting more and more popular.
The interpretation is the work of Hugh Everett III a graduate
student at Princeton at the time he came up with his idea which
he originally called the "relative state" formulation.
Everett's idea is simply that the wave function
never collapses. This puts the Schrödinger's cat thought experiment
on steroids. Not only is the cat in both the alive and dead state
at the same time, the scientist conducting the experiment would
also be split into two with one seeing a dead cat and the other
a live one. This splitting just doesn't pertain to the one "cat"
experiment, however, but to every possible outcome of a quantum
event for every particle in the universe. According to this interpretation,
the universe is continually splitting into infinitely different
versions every moment like a giant branching tree. There are parallel
universes which differ only slightly from ours and others that
vary wildly.
In fact, if you follow this interpretation out
to its logical conclusion, everything that is possible, no matter
how improbable, does exist in some version of the universe. In
one you are the President of the United States. In another, you
are in prison for mass murder. As strange as this seems, the idea
that everything exists, however, has been cited as one of the
interpretations strongest supporting ideas. Cosmologist Max Tegmark,
who has created a hierarchy of multiple universe levels based
on this idea, argues that it is much simpler to describe a set
of universes (sometimes called a multiverse) where everything
exists, rather than a single universe with particular rules. "A
common feature of all four multiverse levels is that the simplest
and arguably most elegant theory involves parallel universes by
default. To deny the existence of those universes, one needs to
complicate the theory by adding experimentally unsupported processes
and ad hoc postulates: finite space, wave function collapse and
ontological asymmetry. Our judgment therefore comes down to which
we find more wasteful and inelegant: many worlds or many words."
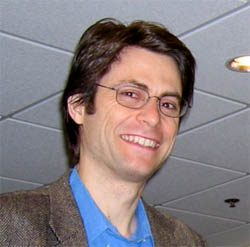
Max Tegmark
is a cosmologist that has come up with a hierarchy of
muliple universes.
|
The many worlds interpretation also solves one of
the most difficult philosophical questions for anybody contemplating
building a time machine. If there is only one universe using a
time machine, to go back in time and kill your grandfather would
create a paradox. This isn't true with a many worlds multiverse.
Killing your grandfather would simply spawn an alternate history
in which you never appear. On another branch of history, your
grandfather would have lived and you would be born. If you returned
to your original branch your grandfather would still be alive.
If you remained in the alternate history where you killed your
grandfather you would be an alien entity with no past.
There are other interpretations of quantum physics
other than Copenhagen, Bohm and Many Worlds. However, all of them
seem to have some form of "weirdness" attached to them. At this
point physicists are still arguing about which interpretation,
if any of them, are correct. The solution will probably have to
wait till some clever physicists comes up with an experiment to
prove or disprove some of these interpretations.
For our conclusion, however, let's move back from
ideas that cannot yet be tested to an experiment that has been
duplicated already in several laboratories. It's one of the most
beautiful experiments ever created: The delayed choice quantum
eraser.
The
Delayed Choice Quantum Eraser Experiment
In the original double slit experiment we noted
if you obtained the "which way" information that told you which
slit the photon passed through the interference pattern disappeared
because now the photon starting acting like a particle instead
of a wave. The delayed choice quantum eraser experiment, originally
done by Yoon-Ho Kim, R. Yu, S.P. Kulik, Y.H. Shih, and Marlan
O. Scully is set up like the double slit we saw in part one. However,
just behind the slits (let's label the slits A and B) is positioned
a beta barium borate crystal (BBO). This crystal, when struck
by a photon, can release two lower energy photons that are entangled
(sharing a single wave function). One photon (called the signal
photon) is allowed to go to a detector (let's refer to this as
D0) where its location can be plotted. Both the paths from slit
A and slit B hit the detector D0 so as more and more photons arrive
this information can be used to see if the photon is, or is not,
part of an interference pattern, just like in the original two
slit experiment.
The entangled partner of the signal photon (called
the idle photon) heads off in a different direction. Like the
signal photon it can travel two different paths (A and B), one
from each slit. The experiment is designed so that paths of the
idle photon are much longer than the paths of the signal photon.
The means that by the time the idle photon encounters any optical
equipment the signal photon has already hit and been registered
by the D0 detector. The idle photon first reaches two beam splitters
(We will call them BSA and BSB, one for each path). A beam splitter
is an optical device that has a 50% chance of letting the photon
go through it like a piece of glass and a 50% chance of reflecting
it like a mirror. If the photon reflected by the beam splitter
it encounters one of two detectors depending on whether it was
following path A or B (We will call these detectors D3 and D4).
If the photons pass through the beam splitters they instead hit
mirrors that bounce them to a single final beam splitter. Here
the photons from one path can go through to hit detector D1 or
reflect to hit detector D2. Photons traveling the other path can
do just the opposite going through to D2 or reflecting to D1.

The
set up for the Delayed Choice Quantum Eraser experiment.
Items marked BS are beam splitters, M are mirrors and D
are detectors. (Credit Patrick Edwin Moran
and Wikapedia Commons)
|
The result of this arrangement is that if a photon
is reflected by the first beam splitter it will be detected at
either D3 or D4 and the "which way" information about which slit
it and its entagled partner went through can be obtained. If the
photon passes through the first beam splitters, however, the final
beam splitter mixes the paths up so we know that a photon has
arrived at D1 or D2, but we have no idea which path it and its
partner traveled through the two slit device.
All the detectors are hooked up to a device known
as a "coincidence counter" which matches the arrival and position
of a signal photon at D0 with a idle photon at D1, D2, D3, D4.
The coincidence counter is needed to keep any spurious photons
from affecting the experiment. Since not every photon that hits
the BBO creates an entangled pair, there can be quite a few unentangled
photons that need to be kept out of the final data.
When the data from this experiment is plotted some
very interesting things can be seen. If an idle photon hits D3
or D4 so we can tell which slit it and its partner took and we
can see that it does not participate in an interference pattern.
If the signal photons from D0 are matched with their partners
from D1 and plotted we do see a clear interference pattern because
we don't know which slit the photon went through. We see the same
thing when we plot D0 and D2 because we again have no idea of
which slit the photon came through (though in this second case
the interference pattern has been shifted a bit over from the
D0/D1 pattern because of the optical properties of the last beam
splitter).
Backwards
in Time?
What is amazing about this experiment is that neither
signal photons or the idle photons do anything much different
as far as physical interaction with the lab equipment in either
case of an interference pattern being formed or not. Going back
to the regular double slit experiment it could be argued that
the detector watching the photon going through the slit somehow
physically interacted with it causing it to change from a wave
to a particle. Here the decision of the whether a signal photon
will interfere with itself is made based solely whether the information
about which slit it went through is still available after the
idle photon hits its detector. Since the idle photon hits its
detector significantly after the signal photon has arrived at
its detector, it would appear that the information is being "erased"
and something is going back in time and changing history. Is that
really what is happening?
From a strict Copenhagen interpretation point of
view, probably not. The signal photon is in superposition when
it arrives at the D0 detector. It has both participated in the
interference pattern and not participated in the interference
pattern. When it hits the detector it entangles its wave function
with that of D0 also putting the detector into superposition.
The wave function only collapses into one or the other situations
when the idle photon arrives at its detector and the observation
is complete. Of course what, or who is needed to complete the
observation (man or machine) is still a matter for debate, though
it would seem that the D0 detector by itself does not qualify
as an observer.
For those people who believe in the Many Worlds
interpretation the superposition never collapses and the universe,
including the scientists doing the experiment is split four ways,
one for each possible detector that the idle photon can arrive
at. It would appear to the each of scientists in their own worlds
as if the arrival of the idle its detector had changed the past,
but in reality it would just be an effect caused by the splitting
of the universe four ways.
So this is a small peek at quantum weirdness. At
this point in time scientists are very sure what quantum theory
works, but still argue about what interpretation we should embrace.
Do our observations create the universe around us, or are we just
tiny specs in a multiverse than contains every possible history?
Or is there some other possibility? Nobody knows for sure.
For many years physicists avoided these questions
and the weirdness of quantum physics was, as scientist and writer
J.M. Jauch put it "a kind of skeleton in the closet." Recently
there has been increasing interest in this area and research into
the meaning of quantum physics is starting to become respectable.
Hopefully this will lead to more answers to what seems to be the
universe's most puzzling questions.
Back
to Part 1
Copyright
Lee Krystek 2010. All Rights Reserved.